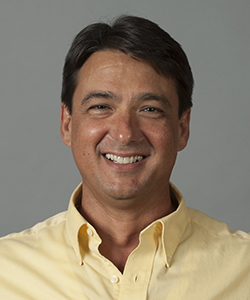
L. Michael Carastro, Jr.
Associate Professor of Chemistry
Education:
- 1994 University of Tampa, B.S. in Biochemistry and Biology
- 2001 University of Miami School of Medicine, Ph.D. in Biochemistry and Molecular Biology
Postdoctoral Training and Faculty Appointments:
- 2001 Postdoctoral Fellow - The Salk Institute for Biological Studies
- 2002 Visiting Postdoctoral Fellow - H. Lee Moffitt Cancer Research Institute
- 2003 Postdoctoral Scholar - Penn State College of Medicine
- 2005 Assistant Professor of Pharmacology - Penn State College of Medicine
- 2007 Collaborator Member - H. Lee Moffitt Cancer Research Institute
Research Interests
Michael Carastro’s research interests are focused on the molecular etiology of human cancers, including the molecular mechanisms that maintain cellular DNA integrity and regulate gene expression. His early research work was in DNA replication/repair, but his dissertation research serendipitously progressed into a novel area of gene expression regulation, called nonsense-mediated mRNA decay (NMD). NMD is a translation-dependent mRNA degradation process that only targets mRNAs containing a translation termination codon recognized as “premature.” Carastro was primary author of a 2002 Nucleic Acids Research article that first reported a connection between cellular DNA replication (DNA polymerase delta) and the nonsense-mediated mRNA decay (NMD) helicase (HUPF1, helicase required for NMD). Since the initial discovery, other groups have published results following this pioneering work and/or cited it in review articles, including Current Opinion in Genetics and Development (Culbertson & Leeds, 2003), Nature Reviews Molecular Cell Biology (Maquat, 2004), Molecular Microbiology (de Pinto et al, 2004), Journal of Cell Science (Maquat, 2005), Molecular and Cellular Biology (Whittmann et al, 2006), Current Biology (Azzalin & Lingner, 2006), and Cell Cycle (Azzalin & Lingner, 2006).
In recent years, Carastro developed and directed two human cell culture-based prostate cancer (PCa) research projects: (1) epigenetic regulation of the TP73 tumor suppressor gene promoter and p73 protein isoform balance, and (2) molecular studies of cellular gene expression changes induced by exposure to Polyphenon E, a proprietary, standardized green tea extract that is rich in polyphenols and caffeine-free. With my research colleague, Dr. Jong Park (Moffitt Cancer Center), we investigated the molecular role of a dinucleotide polymorphism (DNP) in the TP73 tumor suppressor gene. This TP73 DNP project resulted in a peer-reviewed research article (Carastro et al, 2014). The essence of these published findings was the detection of a statistically significant decreased risk for aggressive PCa, as well as altered cellular p73 protein isoform levels, TAp73 and deltaNp73. Also, we worked to develop LC-MS proteomic methodologies, in consultation with the Moffitt Proteomics Core, to quantitatively detect and discriminate between the two p73 protein isoforms. Recently, we quantitatively analyzed the CpG methylated positions within the TP73 gene promoter using pyrosequencing methodologies. We have developed comprehensive, quantitative data that is consistent with a direct relationship between the degree (%) of methylation at specific CpG sites within the p73 gene promoter and the metastatic potential of PCa human cell lines studied. Our TP73 CpG promoter methylation studies are the subject of current research in my laboratory at UT.
Currently, Carastro’s laboratory is conducting molecular oncology studies using human PCa cell cultures treated with Polyphenon E (Poly E) in order to detect gene expression changes related to Poly E treatment of human PCa cells. Poly E is a To date, we have used three PCa cell lines (and normal, primary prostate cells) to generate DNA microarray data, as well as qRT-PCR and western blotting data. Some of these data using PC-3 PCa cells were recently published (Carastro et al, 2022) and included five UT undergraduate co-authors.